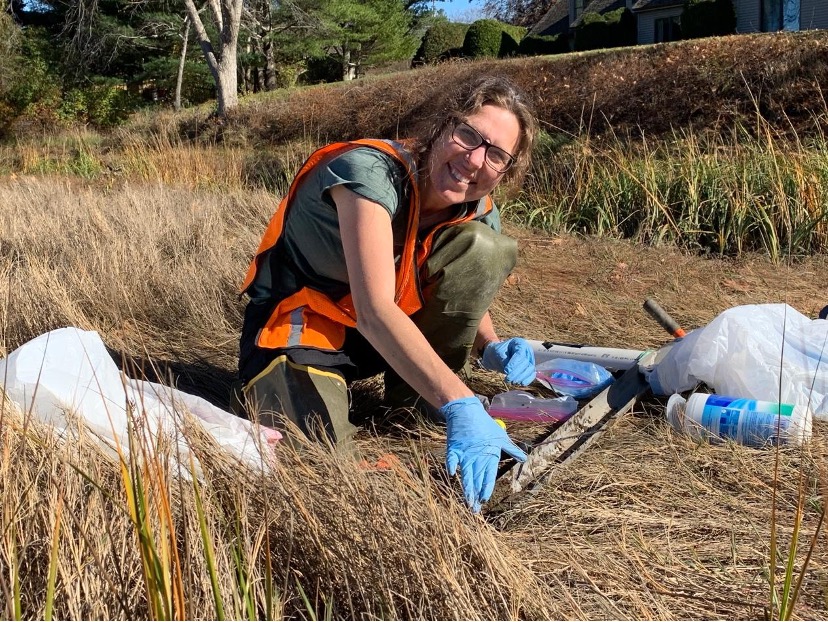
Heather Richard, UMaine MARINE Seed Grant Recipient
Heather grew up in Gorham, Maine and received her undergraduate degree in Biology with a concentration in Evolution and Ecology at Clark University. She worked as a naturalist and educator for nearly a decade in Maine, West Virginia, Florida and California. She received a Master’s of Science in 2016 from San Francisco State University where she studied metal sorption on biofilm coating microplastics at Estuary and Ocean Science Center in Tiburon, CA. After moving back to Maine and gaining more experience as an educator, aquaculture technician and research coordinator in the mid-coast area, she now attends the University of Maine in pursuit of her PhD and lives in Bangor with her cat Boo.
Growing up in Southern Maine, I assumed I knew a thing or two about salt marshes. I’d helped to lead birdwatching trips within marsh habitats, I went canoeing through the Scarborough Marsh just for fun and I took a class as an undergraduate student where we read a book by Dr. Mark Bertness, a salt marsh ecologist whom I admired. So I knew about marshes. Or so I thought. I just never thought I’d study them. For my Master’s degree, I studied in the lab of one of the first researchers to identify plastic pollution in the marine environment. Beginning in 2012, I worked to understand how marine microorganisms colonize very small plastic particles (> 5 mm in diameter) and alter the surface properties which determine the various types of pollutants that adhere to the surface. I loved doing this work, but I was surprised that the microbial life and not the plastic pollution itself sustained my interest in an enduring way. I wanted to learn everything I could about the ways these life forms were interacting with and influencing the impacts of human activities, but I was challenged by the scientific tools I had available to study tiny organisms such as bacteria, algae and fungi.
I moved back to Maine in 2016 and had the great fortune of working for some incredible marine-focused organizations in the areas of education, aquaculture and research. I gained insight and understanding into the issues that are specifically important to Maine but are also globally relevant. Diversifying the harvest of marine resources, understanding the impacts of climate change and detecting and reducing coastal pollution have become areas of great interest for me, and I was excited to learn that the University of Maine’s environmental DNA Program was conducting research relevant to all of these areas. Environmental DNA, or eDNA research utilizes the ability to collect and sequence DNA present in air, water or soil. By doing this, we can learn which species have been present recently in the area and even learn which genes are present so we can better understand what biologic functions are occurring. I wondered if it was possible to improve the health of marine habitats by studying the microbial DNA within that habitat, similar to how we can improve human health by studying gut microbiomes. I applied and was accepted into the NSF EPSCoR RII Track-1 Maine-eDNA program in the fall of 2021 as a PhD student in the lab of Dr. Andy Rominger whose work focuses on large-scale data analyses in the field of Ecology and Evolution.
Together, Andy and I developed a few ideas which we hoped would help with issues relevant to Maine communities as well as the broader research community. Blue carbon is a term that refers to carbon stored within marine habitats, especially vegetated habitats like seagrasses, marshes and mangroves which can sometimes have the ability to store more carbon per square meter than most terrestrial habitats because of the way sediment gets carried in water and trapped within the vegetation structure. I have always loved kayaking along areas with seagrass beds, so I thought it would be interesting to find out which microbial species were indicators of healthy seagrass areas capable of burying lots of carbon and which species indicated more degraded ecosystems. When Dr. Kate Ruskin proposed that we apply for a seed grant with the University’s MARINE program and study the microbial communities above and below restrictions in salt marshes, I thought, “Sure! But what the heck is a restriction?”
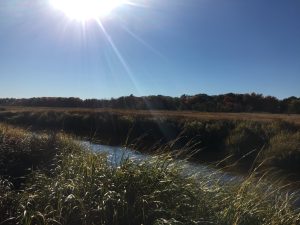
A hydrologic restriction is a bridge or road that crosses a stream or river. These can, to varying degrees, alter the natural flow of water so that water is partially prevented from flowing from one side to another. Water can become impounded on one side of the restriction, or restrictions could cause a naturally occurring wetland to dry out. In salt marshes, these creeks are tidally influenced, meaning that not only is the water flow altered, but also the salinity regime within the habitat is altered. Impounding fresh water upstream while keeping salt water out is one way restrictions can turn sensitive saltmarsh habitat into a soupy wetland filled with cattails or make it vulnerable to the invasive species Phragmites australis. An impounded freshwater wetland can be a place where methanogenic bacteria thrive and as a by-product of their special metabolism produce methane which is a greenhouse gas many times more potent than carbon dioxide in its ability to warm the planet. Alternatively, if a restriction prevents water from entering upstream habitats, the soil dries out and oxygen enters into what was once an anoxic environment. This increases microbial metabolism and thus removes the stored carbon by releasing it as carbon dioxide gas.
Of course, carbon storage is just one of the many important ecosystem services salt marshes provide. They take pollutants out of the water, they nourish and protect young marine life, they protect coastal communities from storm surge and flooding. How habitat managers decide which marshes to focus on for restoration can be a very complex and challenging problem. We asked, can environmental DNA be a useful metric to understand the ecological and carbon sequestration impacts of hydrologic restrictions? If so, can we use this research to inform restoration decisions and/or improve our carbon accounting in Maine’s blue carbon habitats? In January of 2022 we were awarded the research funds and we set to work implementing a pilot study to better understand the interplay between blue carbon, microbes and salt marsh restrictions.
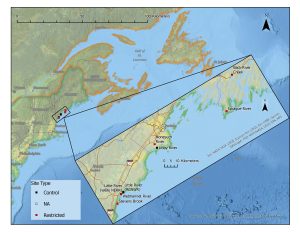
We took nearly 100 sediment cores along 7 different salt marsh creeks from Wells to Phippsburg, ME. We did sample in two unrestricted stretches of marsh as a control comparison, but most of these creeks had restrictions which we centered our sediment sampling around, sampling 50, 150 and 250 meters above and below the restriction. We took cores in low marsh areas close to the creek where saltmarsh cordgrass (Sporobolus alterniflorus, A.K.A Spartina alterniflora) grows, as well in the high marsh where saltmarsh hay (Sporobolus pumilus, A.K.A Spartina patens) is abundant. Our sediment cores were taken in such a way that we could take the section from 5-15 cm and the section from 35-45 cm and split these sections into two subsamples, one for DNA extraction to identify bacterial and fungal communities present in the two depths and the other for physicochemical analysis, measuring carbon content, nutrients, pH and other possibly important variables.
We are still in the process of working through the analysis of these samples, but so far we have learned many lessons about how we might design a future study and achieved an understanding of the bacterial and fungal communities present in these samples which we can use to infer and compare the biological functions that are occurring above and below marsh restrictions. Because species may be present but inactive, the presence of certain species doesn’t necessarily equate to the biological activity of the species, but it is a good starting point with which to ask deeper questions. Once we have fully understood the outcomes of this study, we will undoubtedly have more questions to ask. Does what we found apply to salt marshes in different geographic regions? How do our findings help improve the knowledge of carbon coming in and out of marshes in the state of Maine? How might sampling at different times of the year or under future climate scenarios change microbial composition and activities? It is our hope that this pilot study will provide a strong foundation for answering these questions and many more.
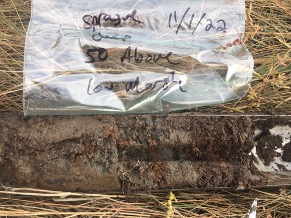
In the future, there will be financial incentive to monetize blue carbon habitats for carbon credits, and the social and ethical implications of how this plays out will be felt throughout Maine’s coastal communities. Funding conservation projects with carbon credits could be a really great thing, but the science and ethics behind it will have to be top notch. I have a few more years to go before I am finished with my PhD, and I can’t wait to get back out into these important coastal ecosystems and continue the work of understanding how they function so we can manage them in ways that maximize the benefits they provide.